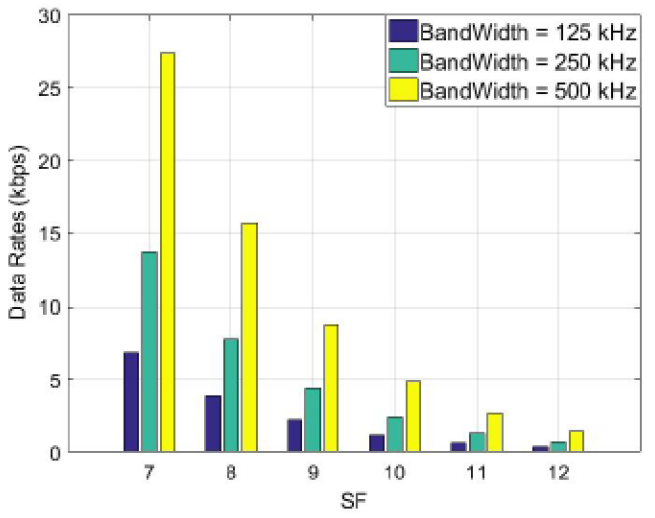
DOI:
https://doi.org/10.14483/23448393.18861Published:
2022-04-25Issue:
Vol. 27 No. 2 (2022): May-AugustSection:
Sección Especial: Mejores artículos extendidos - WEA 2021Data Acquisition with LoraWAN IoT Technology to Monitor Bio-Inspired Wind Turbines in Rural Areas of Cundinamarca
Adquisición de datos con tecnología IoT LoraWAN para monitoreo de turbinas eólicas bio-inspiradas en zonas rurales de Cundinamarca
Keywords:
IoT, LoRaWAN, MCU, HTZ Communications (es).Keywords:
IoT, LoRaWAN, MCU, HTZ Communications (en).Downloads
References
ICCA, “Energías renovables,” 2015 [Online]. Available: http://repiica.iica.int/B3661e/B3661e.pdf
J. Espitia and P. Molina, “Economía colombiana y coyuntura política: La situación del sector eléctrico” [Online]. Available: https://unipacifico.metabiblioteca.org/cgi-bin/koha/opac-detail.pl?biblionumber=11604
J. A. G. Moreno, C. L. T. Rodríguez, and R. A. P. Suesca, “Hybrid electric energy generation like alternative for not inte connected zones,” Ing., vol. 12, no. 1, pp. 57-63, 2006. https://doi.org/10.14483/23448393.2100
L. A. Arias Barragán, E. Rivas Trujillo, and F. Santamaría, “Agente integrador de recursos energéticos distribuidos como oferente de energía en el nivel de distribución TT,” Ing., vol. 22, no. 3, pp. 306-323, 2017. https://doi.org/10.14483/23448393.10986 DOI: https://doi.org/10.14483/23448393.10986
C. Bordons, F. García-Torres, and L. Valverde, “Gestión óptima de la energía en microrredes con generación renovable,” Rev. Iberoam. Autom. Infor. Ind., vol. 12, pp. 117-132, 2015. https://doi.org/10.1016/j.riai.2015.03.001 DOI: https://doi.org/10.1016/j.riai.2015.03.001
S. Madakam, R. Ramaswamy, and S. Tripathi, “Internet of Things (IoT): a literature review,” J. Comput. Commun., vol. 3, no. 5, pp. 164-173, 2015. https://doi.org/10.4236/jcc.2015.35021 DOI: https://doi.org/10.4236/jcc.2015.35021
J. M. G. Álvarez and J. C. G. Targarona, “Generación eólica empleando distintos tipos de generadores considerando su impacto en el sistema de potencia,” DYNA, vol. 78, no. 169, pp. 95-104, 2011. https://revistas.unal.edu.co/index.php/dyna/article/view/20070
J. M. Aranda, “Diseño y evaluación del desempeño de una red de comunicaciones para medición inteligente en Network Simulator–2,” Ing., vol. 20, no. 1, pp. 2–12, 2015. https://doi.org/10.14483/udistrital.jour.reving.2015.1.a02 DOI: https://doi.org/10.14483/udistrital.jour.reving.2015.1.a02
R. Bikmetov, M. Y. A. Raja, and T. U. Sane, "Infrastructure and applications of Internet of Things in smart grids: A survey," presented at 2017 N. Am. Power Symp. (NAPS), Morgantown, WV, USA, Sept. 17-19, 2017. https://doi.org/10.1109/NAPS.2017.8107283 DOI: https://doi.org/10.1109/NAPS.2017.8107283
A. F. Arciniegas M., D. E. Imbajoa R., and J. Revelo F., “Diseño e implementación de un Sistema de Medición Inteligente para AMI de la microrred de la Universidad de Nariño,” Enfoque UTE, vol. 8, no. 1, pp. 300-314, Feb. 2017. https://doi.org/10.29019/enfoqueute.v8n1.136 DOI: https://doi.org/10.29019/enfoqueute.v8n1.136
K. Mekki, E. Bajic, F. Chaxel, and F. Meyer, “A comparative study of LPWAN technologies for large-scale IoT deployment,” ICT Express, vol. 5, no. 1, pp. 1-7, 2019. https://doi.org/10.1016/j.icte.2017.12.005 DOI: https://doi.org/10.1016/j.icte.2017.12.005
A. Manrique, L. Buitrago, and J. Hernández, “Redes LoRaWAN. Revision de componentes funcionales en aplicaciones IoT,” Research Paper, Fac. Tec., Univ. Dist. Francisco José de Caldas, Bogotá, Col., 2019. http://hdl.handle.net/11349/22411
U. Noreen, A. Bounceur, and L. Clavier, “A study of LoRa low power and wide area network technology,” presented at 2017 Int. Conf. Adv. Tech. Sig. Image Proc. (ATSIP), Fez, Morocco, May 22-24, 2017. https://doi.org/10.1109/ATSIP.2017.8075570 DOI: https://doi.org/10.1109/ATSIP.2017.8075570
W. A. Porras-Calderón and O. Y. Salah-García, “Implementación de una Red LPWAN de Sensores para el Monitoreo de Condiciones Ambientales,” Met&Flu, 2019, [Online]. Available: https://www.cdtdegas.com/images/Descargas/Nuestra_revista/MetFlu14/9_RedLPWAN.pdf
LoRa® Alliance Technical Marketing Workgroup, “A technical overview of LoRa ® and LoRaWAN TM What is it?,” 2015 [Online]. Available: https://lora-alliance.org/resource_hub/what-is-lorawan/
RAKwireless Technology, “Documentation center RAK7258 WisGate Edge Lite datasheet overview description”, https://docs.rakwireless.com/Product-Categories/WisGate/RAK7258/Supported-LoRa-Network-Servers/#aws-iot-core-for-lorawan (accessed Jan, 2022).
RAKwireless Technology, “RAK811 Evaluation Board Quick Start Guide”, https://docs.rakwireless.com/Product-Categories/WisDuino/RAK811-Evaluation-Board/Quickstart/ (accessed Jan, 2022).
Hackster.io, “Using LoRaWAN end devices on The Things Network.” https://www.hackster.io/nootropicdesign/using-lorawan-end-devices-on-the-things-network-206a86 (accessed May 11, 2021).
J. C. Merino, “Deployment and evaluation of an on-site LoRaWAN network based on The Things Stack version 3,” Esc. Téc. Sup. Ing. Ind. Telec., U. Cantabria, Cantabria, Spain, Oct. 2019. https://repositorio.unican.es/xmlui/bitstream/handle/10902/17087/420136.pdf?sequence=1&isAllowed=y
How to Cite
APA
ACM
ACS
ABNT
Chicago
Harvard
IEEE
MLA
Turabian
Vancouver
Download Citation
Recibido: 30 de diciembre de 2021; Revisión recibida: 18 de noviembre de 2021; Aceptado: 9 de febrero de 2022
Abstract
Context:
The energy crisis is a global problem. In Colombia, research aimed at the efficient use of renewable energies is being conducted. For example, in the case of bio-inspired wind generators, a mo- nitoring system is required to observe the output variables (voltage and frequency).
Method:
LoRaWAN technologies are used to deploy the wireless link since the generators are separated from the base station. In addition, the Gateway coverage area is simulated with HTZ Communications software for future smart grid improvements.
Results:
The implemented system allows constant monitoring over a long period of time and stores its information in online, open-source databases, which allows remotely visualizing the status of the network, with a total of 3 samples per minute and a range of up to 3,47 km.
Conclusions:
The data acquisition system can be implemented for remote monitoring. Moreover, since it is a low power system, it can be utilized in non-interconnected areas, as well as for storing data for up to 6 months. However, for the characterization of a model of wind generators, it is advisable to modify the system so that the sampling time is lower.
Keywords:
IoT, LoRaWAN, MCU, HTZ Communications..Resumen
Contexto:
La crisis energética es una problemática global. En Colombia se están realizando investigaciones para el aprovechamiento eficiente de energías renovables. Por ejemplo, en el caso de generadores eólicos bio-inspirados, se requiere un sistema de monitoreo para observar variables de salida (voltaje y frecuencia).
Método:
Se utilizan tecnologías LoRaWAN para el despliegue del enlace inalámbrico, ya que los generadores están separados de la estación base. Además, la zona de cobertura del Gateway se construye en el software HTZ Communications para futuras mejoras en la red inteligente.
Resultados:
El sistema implementado permite realizar un monitoreo constante por periodos de tiempo prolongados y almacena su información en bases de datos online de código abierto, lo cual permite visualizar el estado de la red de forma remota, con un total de 3 muestras por minuto y un alcance de hasta 3,47 km.
Conclusiones:
El sistema de adquisición de datos puede ser implementado para monitoreo remoto. Adicionalmente, dado que es un sistema de baja potencia, puede utilizarse en zonas no interconectadas, así como para almacenar datos hasta por 6 meses. Sin embargo, para la caracterización de un modelo de generadores eólicos, es aconsejable modificar el sistema para que el tiempo de muestreo sea menor.
Palabras clave:
IoT, LoRaWAN, MCU, HTZ Communications..Introduction
Renewable sources in the world are a type of solution to the energy and environmental problems that have been arising in recent decades 1. Colombia, a developing country, has been aware and working to mitigate this global problem, given that water is its most used resource for energy pro- duction, followed by power plants 2. Therefore, in recent years, Colombia has been interested in gradually increasing the use of alternative energies, as is the case of smart grids 3, offering a solu- tion to supply energy to remote or non-interconnected rural areas (ZNI), which cannot be supplied via the main power plants of the country.
Smart grids are a set comprising a system of loads, generators, and energy storage that can be ma- naged in isolation or connected to the rest of the electrical grid 4, a concept that was introduced by Lasseter in 2002 5. Smart grids can base their operation on the use of air currents, but the latter have disadvantages in the production of electrical energy, since their generation is intermittent. For this reason, there is a field of development aimed at increasing the efficiency of wind generators, wherein it is necessary to implement monitoring systems in smart grids, which may be based on the Internet of Things (IoT).
IoT is an open network of intelligent objects with the ability to share information, data, and resources, reacting to and acting on situations and changes in their environment 6. In the case of smart grids, IoT is widely used to monitor the behavior of a system. Moreover, it is important J. A. Quintero • H. G. Parra Peñuela • E. E. Gaona to mention that this technology allows remóte monitoring of the variables resulting from power generation, which supports decision-making 7, 8.
Methodology
In this work, a system for the acquisition and monitoring of voltage and frequency variables is developed in order to evaluate the performance of the energy use produced by bio-inspired wind tur- bines. Different phases are implemented: selection of the IoT technology, materials, development, and implementation. The following sections describe each of the stages involved in the process (Fig. 1).
Figure 1: Phases of the elaboration of the data acquisition system to monitor energy production
Selection of the IoT technology
In the exploration stage, a low consumption system was sought, with the purpose of not wasting the energy produced in a high-power communication system. Moreover, this selection should not affect the coverage area, since some requirements of the project establish that this network should be available for future updates in the project and new applications that may involve constant monitoring. Likewise, a low-cost system was needed.
The concept of the IoT is closely related to smart grids in such a way that these can interact between several devices with minimal human intervention; the combination of these two systems is known as smart grid 9, which has applications in data processing and decision-making, thus allowing to maximize the energy produced, a situation that has proven to be problematic in the context of renewable energies 10.
Additionally, the searches conducted on IoT and its implementation in a smart grid network found that there are several key parameters that define what type of LPWAN technology could be imple- mented in the project. Among them are bandwidth, working frequency, distance, and licensed or unlicensed band, among others (Table I) 8. After reviewing the implementation requirements, the LoRaWAN technology was chosen since it works in an unlicensed band and has a payload higher than Sigfox, unlimited number of messages, 128-bit communication encryption, and low power consumption.
Table I: Overview of LPWAN technologies: Sigfox, Lora, andNB-IoTx 11
LoRa is a low power private wireless communications protocol that operates in the physical la- yer of the OSI reference model. It has working frequencies in unlicensed bands and uses spread spectrum modulation (CSS, Chirp Spread Spectrum). Additionally, the fact that it is based on chirp technology allows high tolerance to interference and high sensitivity to receive data up to -168 dB. This is due to transmission parameters such as channel frequency, bandwidth (BW), spreading factor (SF), and chirp rate (CR), as well as a greater range or higher transmission speed, which is required in the development of the project 12.
Bandwidth (BW). The lora modules have three configurations for the 125, 250, and 500 kHz bandwidths, modifying the data transmission between two devices. In addition, the transmitter module sends the spread data at a chirp rate equal to the system’s bandwidth in chirps per second per Hz, so the 250 kHz bandwidth corresponds to a speed of 250 kcps 13.
Spreading factor (SF). It is a factor that allows configuring the range that a radio link can have between the transmitter and the receiver device. This parameter has a range between 7 and 12, and the higher the SF, the greater the range that the signal can reach, which, however, decreases the data rate and vice versa. Fig. 3 shows how the SF affects the transition speed (Fig. 2a) 14.
Figure 2: a) Relationship between data rate and SF expansion factor; b) relationship between CR and effective data rate with SF=7 13
Figure 3: LoRaWAM network architecture 15
Code Rate (CR). LoRa networks are connection-oriented technologies, and the modulation adds forward error correction (FEC) to each data transmission. This FEC can be configured through the CR parameter in LoRa modules, offering CR settings between 0 and 4, where CR = 0 means no FEC. LoRa uses code rates of 4/5, 2/3, 4/7, and 1/2. This means that, if CR is denoted as k = n, where k represents useful information, and the encoder generates n number of output bits, then n - k will be the redundant bits 13. This coding considerably decreases the transmission rate (Fig. 2b).
LoRaWAN. It is a low power and wide area network protocol designed to interconnect IoT devi- ces based on a star topology between the end devices and the Gateway (Fig. 3). Moreover, LoRa- WAN also offers authentication, confidentiality, and integrity services through AES128 encryption, so that the information can only be interpreted by the ends of the link 15.
Once the technology to be used had been selected, research was conducted on the devices that were in the market and which of them could be used for implementation in a rural area of Cundinamarca, knowing that there were limitations in terms of equipment costs. There are modules developed by the RAK company that can be used in embedded systems, which is why one such module was chosen, thus allowing the implementation of different libraries for communication between the embedded systems.
Materials
Gateway LoRaWAN technology
RAK7258 WisGate Edge Lite (Fig. 4a) is a full 8-channel gateway with built-in Ethernet con- nectivity for easy configuration. In addition, there is an on-board Wi-Fi feature that allows it to be easily configured through the default Wi-Fi AP mode. Furthermore, the Gateway has line-of-sight (LoS) ranges of up to 15 km, whereas, for highly urbanized environments, it can cover more than 2 km. It is a perfect solution for any LoRaWAN use case scenario 16.
Figure 4: Physical structure of the RAK7258 Gateway 16; b) RAK811 module evaluation board 17
Gateway Features:
-
8 channels - RX Sensitivity: -139 dBm (min.)
-
TX Power: 27 dBm (max.)
-
Frequencies: EU433 / CN470 / EU868 / US915 / AS923 / AU915 / IN865 / KR920
This gateway is responsible for communicating with end devices, so it must have a high sensiti- vity and an indoor configuration to be used when the line of sight is less than 15 km. Additionally, it has 8 channels, which allows having 8 remote nodes connected simultaneously, thus allowing the project to be scalable for future applications.
WisDuino RAK811 module evaluation board
The WisDuino (RAK811) evaluation board is a development board that comes with the Uno embedded system structure (Fig. 4b). It has a built-in MCU that allows it to function as a standalo- ne solution or as a socket of an embedded system. This board is very suitable for fast development and testing. In addition to the integrated USB-UART port and conversion, it can also be configured with AT commands 17.
Features of the RAK811 LoRa module - It supports LoRaWAN or LoRaP2P (point-to-point link)
-
-Low power module for battery-powered remote applications
-
High receiver sensitivity (-146 dBm)
-
Transmitter power between 14 and 20 dBm
-
Coverage of up to 15 km in suburbs and up to 5 km coverage in urban areas
-
UART communication 17
This module is responsible for wirelessly sending and receiving information from an embedded system to a Gateway. Therefore, this device allows configuring the characteristics of SF, CR, BW, and use frequency, thus allowing to have a greater range or higher transmission speed in the link.
The Things Network (TTN)
The Things Network is a global, open, decentralized, free IoT network. This service allows com- munication between things without the need for a 3G, 4G, or WiFi connection. It was created in 2015, with the aim of facilitating the design and manufacture of networks for the Internet of Things. In addition, it offers HTTP and MQTT integrations, as well as integration with applications deve- loped in Java, Node.js, or Go (Fig. 5) 19.
Figure 5: Network design with TTN 18
Implementation
The development of the project was carried out with the prototype of a system that allows re- ceiving the information of the variables generated by the use of wind energy in wind generators, namely the frequency and the RMS voltage of the generator. Fig. 6 shows the parameters of the system.
Figure 6: Data acquisition system for wind generators
The maximum values of the variables are in the order of 10 Hz and 45V RMS, so signal condi- tioning is necessary to enter the embedded system (Fig. 7). The voltage ratio that must enter the embedded system was calculated (Eq. 1); a ratio close to 13:1 was estimated, thus avoiding satu- ration in the embedded system. Additionally, an optocoupler was added to measure the frequency through interruptions in the embedded system.
Figure 7: Conditioning circuit
Once this stage was completed, we proceeded with information processing in the embedded sys- tem, identifying how an algorithm that allows precision without sacrificing speed can be implemented to later send the information to the RAK811 module, so the latter can transmit the data wirelessly to the Gateway (Fig. 8).
Figure 8: Pseudocode of the embedded system algorithm
Afterwards, the RAK811 module communicates with the embedded system. Unfortunately, the board can only be connected by cables and not by placing the socket, since this causes short circuit errors. Therefore, it is necessary to remove some resistors from the board, which is why we decided to use cables in order to enable communication between the microcontroller and the module 19.
Subsequently, the TTN platform was implemented (version 2) to establish the application para- meters set for the initial configuration of the node, namely the device EUI, the application EUI, and the app key. With this configuration, the information is encrypted and can only be processed by the ends of the communication node and its application in the cloud (Fig. 9a).
Finally, integration with The ThingSpeak, an open-source database (Fig. 9b), was performed. The ThingSpeak is a free database that allows storing up to 3 million samples and is ideal for applications focused on IoT. However, the database only allows receiving data every 20 seconds, which limits the continuous analysis of data, although it is recommended for applications involving long periods of time, as it can store up to six months of data for the four variables for later analysis.
Figure 9: a) Device parameters for application; b) integration of TTN with The ThingSpeak
Tests
Since the project was developed during the COVID-19 pandemic, indoor tests were performed, acquiring data from the power grid in Colombia, with the following standard values: a voltage of 110-120 V RMS (Fig. 10a) and a frequency of 60 Hz (Fig. 10b). The following graphs show the behavior of the power grid, considering the variations stemming from uncertainty in data collection. These data were conditioned and captured by the embedded system to be subsequently sent to the server of The Things Network.
Figure 10: left) RMS voltage vs. time; right) frequency vs. time
As it can be observed, the system shows small variations in the main voltage because it must average several voltage samples on the embedded system pin, in addition to the requirements. The system must perform the reading of two wind turbines, which has a higher computational cost, thus affecting the information transmission speed.
On the other hand, the integration of ThingSpeak does not store all the data sent by the embedded system, which affects the quality of the signal. This is due to the fact that the communication link with the TTN server loses frames due to writing limitations, in addition to losing information from the TTN server to the ThingSpeak integration, which also has limitations.
Finally, tests were performed with a simulation software (HTZ Communications), which allows finding the coverage area for wireless links such as IoT. By running this simulation, it was found that the area where the application was deployed can be considered as a small town surrounded by wooded areas (green), avenues (orange), and suburban areas (blue). This can affect the range of the link through attenuations contemplated by the software (Fig. 11).
Figure 11: Installation point of the energy production monitoring system in a prototype of wind generators
This simulation was performed through calculations regarding free space losses and interference in the deployment area using the Okumura-Hata propagation method (Fig. 12). It was observed that the maximum distance between a remote node and the Gateway is 3,47 km, whereas the minimum is 2,44 km with -120 dBm. This is due to the losses that exist due to small, wooded areas, adja- cent buildings, and surrounding crops, which reduce five-fold the coverage area specified by the manufacturer. Additionally, the models are an approximation to reality; they are subject to small variations that must be taken into account by variables not considered in the implemented method. However, these do not affect the estimation of the coverage area.
Figure 12: IoT system coverage zone
Discussion
The data acquisition and monitoring system implemented in bio-inspired wind generators allows storing information and displaying it simultaneously. This allows for remote monitoring of the system, a tool that is essential for applications in non-interconnected rural areas (ZNI). However, by observing the system in operation, it was found that the implemented tools and materials have lia Névíi, a Comcetx >oi mitations that may impair the sampling of variables. Among them is the type of embedded system used, given that it must perform the reading of the variables, information processing, and coding of the frames sent to the node. Therefore, the frame time is proportional to the computational cost of performing each task, thus reducing the transmission speed between the node and the Gateway.
In addition, The Things Network service has limitations regarding the number of frames it can receive from the node, since the integrated system generates frames every 200 ms, and TTN can only process frames every 3 seconds, so it receives 1 frame for every 15 frames, which is equivalent to approximately 7 % of the information. However, a low spread factor (SF7), a 4/5 code rate, and a 125 kHz bandwidth were used, which was initially configured to avoid delays in the wireless link, but modifying some parameters, such as the SF to 12 and a higher bandwidth (500 kHz), allows the LoRa RAK811 module to decrease the transfer rates, which results in more frames arriving successfully and in a greater coverage.
Finally, it was observed that the frames delivered to TTN are not reflected in the database, since one out of five frames were stored in The ThingSpeak database, which is due to the fact that the integration has limitations for storage and allows to store data every 15 seconds. This is detrimental to the monitoring of wind generators; in order to model the behavior of the generators, a system is needed which can store the information faster by means of another type of communication link. However, if one desires monitoring for long periods of time, this system can be recommended.
Conclusions
The design of a data acquisition system for wind generators presented above can have applications in urban, suburban, or non-interconnected areas, since monitoring can be performed from anywhere in the world. Unfortunately, the whole system has a dead time of more than 15 seconds per hour, so it is not recommended. However, it can be recommended for decision-making applications or smart grid monitoring.
Likewise, the prototype can implement another type of integration, different from ThingSpeak, where 4 out of 5 frames sent by The Things Network are lost (80 %). However, the application is recommended for collecting information on the behavior of some variables over a long period of time, sacrificing the time between sample and sample, in addition to being free and easy to deploy.
Regarding hardware, a system can be implemented to improve the sampling quality of the variables, such as dedicated modules for ADC functions, together with an embedded system with concurrency in the processes, which improves the time between frames.
Acknowledgements
Acknowledgements
To the Center for Research and Scientific Development (CIDC) regarding their financial support to project 2-602-608-19, titled Development of bio-inspired active vortex generators for wind turbines in rural areas of Cundinamarca through CFD, by means of project bank call 03-2019.
References
License
Copyright (c) 2022 HECTOR GUILLERMO PARRA PEÑUELA, Elvis Gaona

This work is licensed under a Creative Commons Attribution-NonCommercial-ShareAlike 4.0 International License.
From the edition of the V23N3 of year 2018 forward, the Creative Commons License "Attribution-Non-Commercial - No Derivative Works " is changed to the following:
Attribution - Non-Commercial - Share the same: this license allows others to distribute, remix, retouch, and create from your work in a non-commercial way, as long as they give you credit and license their new creations under the same conditions.