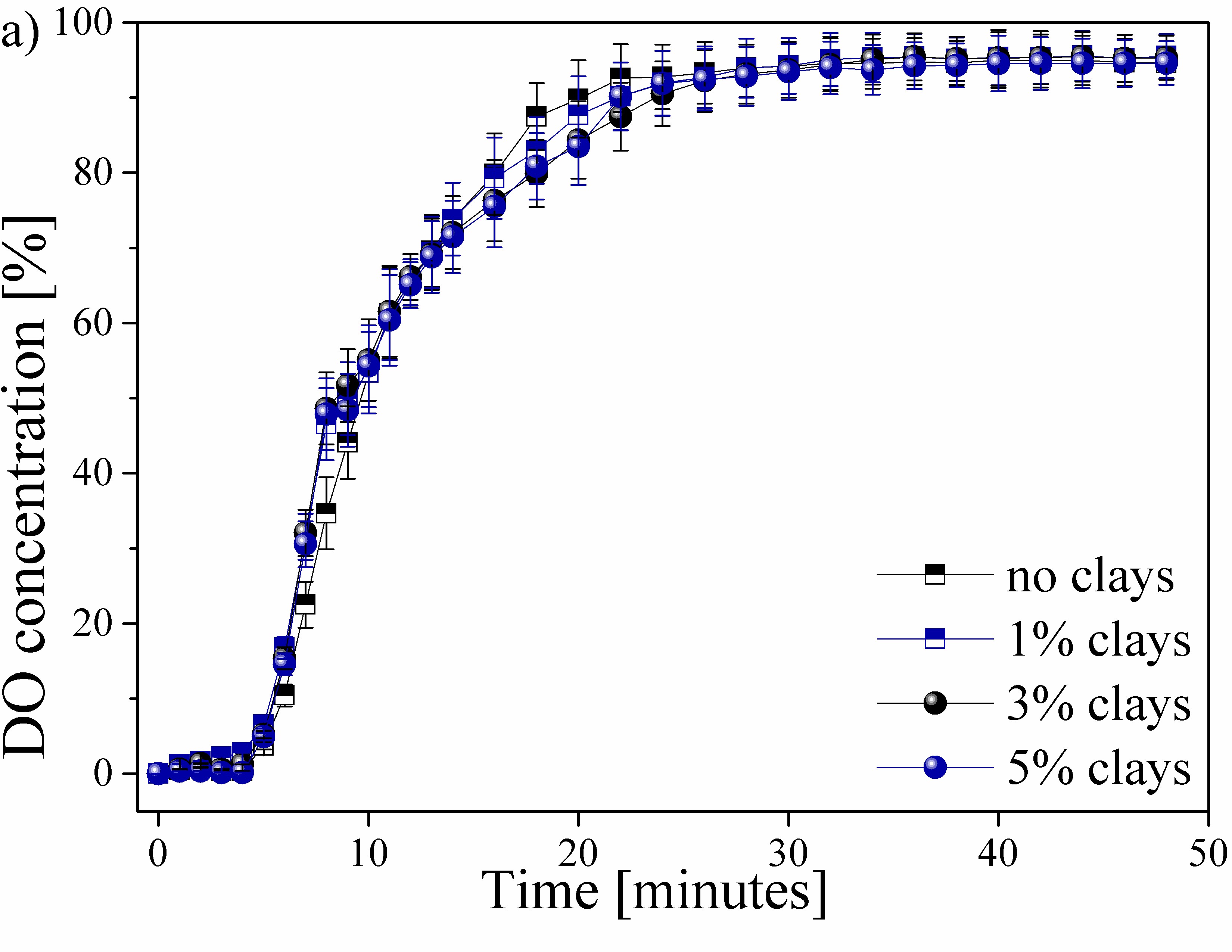
DOI:
https://doi.org/10.14483/23448393.15846Published:
2020-12-19Issue:
Vol. 26 No. 1 (2021): January-AprilSection:
Environmental EngineeringStudy of the Influence of Clays on the Transfer of Dissolved Oxygen in Water
Estudio de la influencia de arcillas en la transferencia de oxígeno disuelto en agua
Keywords:
Dissolved oxygen (DO), diffuse aeration systems, clays, anoxic water (en).Keywords:
Oxígeno disuelto (OD), sistemas de aireación difusa, arcillas, agua anóxica (es).Downloads
References
A. S. Ren, F. Chai, H. Xue, D. M. Anderson, and F. P. Chavez, “A Sixteen-year Decline in Dissolved Oxygen in the Central California Current,” Sci. Rep., vol. 8, no. 1, pp. 1–9, 2018. https://doi.org/10.1038/ s41598-018-25341-8 DOI: https://doi.org/10.1038/s41598-018-25341-8
J. Mueller,W. C. Boyle, and H. J. Popel, Aeration: principles and practice, 1st ed., vol. 11. Boca Raton, FL, USA, CRC Press, 2002. https://doi.org/10.1201/9781420010343 DOI: https://doi.org/10.1201/9781420010343
L. D. Dien, L. H. Hiep, S. J. Faggotter, C. Chen, J. Sammut, and M. A. Burford, “Factors driving low oxygen conditions in integrated rice-shrimp ponds,” Aquaculture, vol. 512, p. 734315, Oct., 2019. https://doi.org/10.1016/j.aquaculture.2019.734315 DOI: https://doi.org/10.1016/j.aquaculture.2019.734315
G. Kumar, C. Engle, T. Hanson, C. Tucker, T. Brown, L. Bott, L. Roy, C. Boyd, M. Recsetar, P. Jeonghwan, and E. Torrans, “Economics of alternative catfish production technologies,” J. World Aquac. Soc., vol. 49, no. 6, pp. 1039–1057, Dec., 2018. https://doi.org/10.1111/jwas.12555 DOI: https://doi.org/10.1111/jwas.12555
E. Durán Herrera and G. Rojas Meza, “Modelación de la transferencia de oxígeno en sistemas de aireación mediante un modelo de dos zonas simplificado,” Cienc. y Tecnol., vol. 24, no. 2, pp. 109–127, 2006. ISSN: 0378-0524
L. Uby, “Next steps in clean water oxygen transfer testing – A critical review of current standards,” Water Res, vol. 157, pp. 415–434, Jun. 2019. https://doi.org/10.1016/j.watres.2019.03.063 DOI: https://doi.org/10.1016/j.watres.2019.03.063
T. W. Brown, C. S. Tucker, and B. L. Rutland, “Performance evaluation of four different methods for circulating water in commercial-scale, split-pond aquaculture systems,” Aquac. Eng., vol. 70, pp. 33–41, 2016. https:// doi.org/10.1016/j.aquaeng.2015.12.002 DOI: https://doi.org/10.1016/j.aquaeng.2015.12.002
J. A. Rojas, Tratamiento de aguas residuales. Teoría y principios de diseño, 3rd ed. Bogotá, Colombia, Escuela Colombiana de Ingeniería, 2010.
V. P. Singh, Entropy theory in hydrologic science and engineering, New York, NY, McGraw Hill Professional, 2015.
D. Carrillo S. Carvajal, J. Coto, V. Salgado, J. Herrera, D. Rojas, and C. Benavidez, “Variación del oxígeno disuelto en el Río Burío- Quebrada Seca, Heredia, Costa Rica, en el periodo 2005 - 2010”, Laboratorio de Manejo del Recurso Hídrico, Universidad Nacional de Costa Rica, Heredia, Costa Rica, 2012. [Online]. Available: http://hdl.handle.net/11056/7468
L. S. Quiroz, E. Izquierdo, and C. Menéndez, “Study of the environmental impact of wastewater discharges on the self-purification capacity in portoviejo river, Ecuador,” Rev. Cent. Azúcar, vol. 45, no. 1, pp. 73–83, 2018. http://centroazucar.uclv.edu.cu/index.php/centro_azucar/article/view/55
H. Muñoz, S. Orozco, A. Vera, J. Suárez, E. García, M. Neria, and J. Jiménez, “Relación entre oxígeno disuelto, precipitación pluvial y temperatura: río Zahuapan, Tlaxcala, México,” Tecnol. y Cienc. del Agua, vol. 6, no. 5, pp. 59-74, 2015. http://www.revistatyca.org.mx/ojs/index.php/tyca/article/view/1170/ 1073
P. Córdova, D. Calderón, and G. Argota, “Medición del coeficiente de transferencia del oxígeno en aguas residuales,” Campus, vol. 23, no. 26, pp. 123–128, Dec. 2018. https://doi.org/10.24265/campus.2018. v23n26.02 DOI: https://doi.org/10.24265/campus.2018.v23n26.02
Y. Ouyang, G. Feng, P. Parajuli, T. Leininger, Y. Wan, and J. N. Jenkins, “Assessment of Surface Water Quality in the Big Sunflower RiverWatershed of Mississippi Delta Using Nonparametric Analysis,” Water, Air, Soil Pollut., vol. 229, no. 11, p. 373, Nov. 2018. https://doi.org/10.1007/s11270-018-4022-8 DOI: https://doi.org/10.1007/s11270-018-4022-8
Y. Ouyang, P. B. Parajuli, G. Feng, T. D. Leininger, Y. Wan, and P. Dash, “Application of Climate Assessment Tool (CAT) to estimate climate variability impacts on nutrient loading from local watersheds,” J. Hydrol., vol. 563, pp. 363–371, Aug. 2018. https://doi.org/10.1016/j.jhydrol.2018.06.017 DOI: https://doi.org/10.1016/j.jhydrol.2018.06.017
S. Shrestha, J. Farrelly, M. Eggleton, and Y. Chen, “Effects of conservation wetlands on stream habitat, water quality and fish communities in agricultural watersheds of the lower Mississippi River Basin,” Ecol. Eng., vol. 107, pp. 99–109, Oct. 2017. https://doi.org/10.1016/j.ecoleng.2017.06.054 DOI: https://doi.org/10.1016/j.ecoleng.2017.06.054
N. Chen, G. Fang, G. Liu, D. Zhou, J. Gao, and C. Gu, “The degradation of diethyl phthalate by reduced smectite clays and dissolved oxygen,” Chem. Eng. J., vol. 355, pp. 247–254, Jan. 2019. https://doi.org/10.1016/j.cej.2018.08.160 DOI: https://doi.org/10.1016/j.cej.2018.08.160
R. Cardoso, I. Pires, S. O. D. Duarte, and G. A. Monteiro, “Effects of clay’s chemical interactions on biocementation,” Appl. Clay Sci., vol. 156, pp. 96–103, May 2018. https://doi.org/10.1016/j.clay.2018.01.035 DOI: https://doi.org/10.1016/j.clay.2018.01.035
INCITEMA, “Análisis de Falla y Evaluación de Materiales,” INCITEMA, 2018. [Online]. Available: https://incitema.com/index.php/analisis-de-falla-y-evaluacion-de-materiales/
N. Guo, C. Thomas, J. Tang, and Q. Tong, “Mapping white mica alteration associated with the Jiama porphyryskarn Cu deposit, central Tibet using field SWIR spectrometry,” Ore Geol. Rev., vol. 108, pp. 147–157, May 2019. https://doi.org/10.1016/j.oregeorev.2017.07.027 DOI: https://doi.org/10.1016/j.oregeorev.2017.07.027
G. Na, G. WenBo, L. Dong, H. YiRu, Z. Long, and G. YaNan, “Alteration zoning model associated with Tibetan Sinongduo epithermal deposit, the continental volcanic areas of Gangdise metallogenic belt,” Acta Petrol. Sin., vol. 35, no. 3, pp. 833–848, 2019. https://doi.org/10.18654/1000-0569/2019.03.13 DOI: https://doi.org/10.18654/1000-0569/2019.03.13
Instituto Colombiano de Geología y Minería, Evaluación geoquímica para geología médica en las zonas asociadas a las fuentes termales del departamento de Boyacá: Sectores Paipa -Iza y Soatá - Guicán. Versión 1.0, Bogotá, Colombia, Ingeominas, 2010.
A. Marsh, A. Heath, P. Patureau, M. Evernden, and P. Walker, “Alkali activation behaviour of un-calcined montmorillonite and illite clay minerals,” Appl. Clay Sci., vol. 166, pp. 250–261, Dec. 2018. https://doi.org/10.1016/j.clay.2018.09.011 DOI: https://doi.org/10.1016/j.clay.2018.09.011
S. Gillot, “Effect of air flow rate on oxygen transfer in an oxidation ditch equipped with fine bubble diffusers and slow speed mixers,” Water Res., vol. 34, no. 5, pp. 1756–1762, Apr. 2000. https://doi.org/10.1016/S0043-1354(99)00323-1 DOI: https://doi.org/10.1016/S0043-1354(99)00323-1
P. R. Cogate, A. A. Beenackers, and A. B. Pandit, “Multiple-impeller systems with a special emphasis on bioreactors: a critical review,” Biochem. Eng. J., vol. 6, pp. 109–144, 2000. https://doi.org/10.1016/S1369-703X(00)00081-4 DOI: https://doi.org/10.1016/S1369-703X(00)00081-4
J. V. Littlejohns and A. J. Daugulis, “Oxygen transfer in a gas–liquid system containing solids of varying oxygen affinity,” Chem. Eng. J., vol. 129, no. 1–3, pp. 67–74, 2007. https://doi.org/10.1016/j.cej.2006.11.002 DOI: https://doi.org/10.1016/j.cej.2006.11.002
A. Bahadori and H. B. Vuthaluru, “Simple Arrhenius-type function accurately predicts dissolved oxygen saturation concentrations in aquatic systems,” Process Saf. Environ. Prot., vol. 88, no. 5, pp. 335–340, Sep. 2010. https://doi.org/10.1016/j.psep.2010.05.002 DOI: https://doi.org/10.1016/j.psep.2010.05.002
Y. Du, F. Chen, L. Zhou, T. Qiu, and J. Sun, “Effects of different layouts of fine-pore aeration tubes on sewage collection and aeration in rectangular water tanks,” Aquac. Eng., vol. 89, p. 102060, May 2020. https://doi.org/10.1016/j.aquaeng.2020.102060 DOI: https://doi.org/10.1016/j.aquaeng.2020.102060
A. Tekile, I. Kim, and J.-Y. Lee, “Extent and persistence of dissolved oxygen enhancement using nanobubbles,” Environ. Eng. Res., vol. 21, no. 4, pp. 427–435, Sep. 2016. https://doi.org/10.4491/eer.2016.028 DOI: https://doi.org/10.4491/eer.2016.028
J. Huang and T. Saito, “Influences of gas–liquid interface contamination on bubble motions, bubble wakes, and instantaneous mass transfer,” Chem. Eng. Sci., vol. 157, pp. 182–199, Jan. 2017. https://doi.org/10.1016/j.ces.2016.05.013 DOI: https://doi.org/10.1016/j.ces.2016.05.013
M. K. Stenstrom, S.-Y. (Ben) Leu, and P. Jiang, “Theory to Practice: Oxygen Transfer and the New ASCE Standard,” Water Environ. Found., pp. 4838–4852, 2006. https://doi.org/10.2175/193864706783762931 DOI: https://doi.org/10.2175/193864706783762931
S. Bun, N. Chawaloesphonsiya, F. Nakajima, T. Tobino, and P. Painmanakul, “Comparative study of local gasliquid hydrodynamics and mass transfer between conventional and modified airlift reactors,” J. Environ. Chem. Eng., vol. 7, no. 4, p. 103206, Aug. 2019. https://doi.org/10.1016/j.jece.2019.103206 DOI: https://doi.org/10.1016/j.jece.2019.103206
J. Lee, “Baseline Mass-Transfer Coefficient and Interpretation of Nonsteady State Submerged Bubble-Oxygen Transfer Data,” J. Environ. Eng., vol. 146, no. 1, p. 04019102, Jan. 2020. https://doi.org/10.1061/(ASCE)EE.1943-7870.0001624 DOI: https://doi.org/10.1061/(ASCE)EE.1943-7870.0001624
D. O. Pino-Herrera, Y. Fayolle, Sylvain Pageot, D. Huguenot, G. Esposito, E.D. Hullebusch, and Y. Pechaud, “Gas-liquid oxygen transfer in aerated and agitated slurry systems with high solid volume fractions,” Chem. Eng. J., vol. 350, pp. 1073–1083, Oct. 2018. https://doi.org/10.1016/j.cej.2018.05.193 DOI: https://doi.org/10.1016/j.cej.2018.05.193
How to Cite
APA
ACM
ACS
ABNT
Chicago
Harvard
IEEE
MLA
Turabian
Vancouver
Download Citation
Visitas
Dimensions
PlumX
Downloads
Recibido: 30 de abril de 2020; Revisión recibida: 6 de agosto de 2020; Aceptado: 18 de agosto de 2020
Abstract
Context:
The lack of dissolved oxygen (DO) in water bodies has become a serious problem for several ecosystems due to drastic changes in their climatic, biological, and physical conditions. The presence of microorganisms, organic matter, and clays, which are carried by landslides and rainwater, affect the process of restoration and transfer of DO in water, thus making it necessary to study how these factors affect the process of DO transfer in water bodies.
Method:
This work is focused on studying the influence of clays during the DO transfer process in water bodies. To this effect, samples of clays from the region of Paipa, Boyacá, were added in 1, 3 and 5% by weight into the samples of clean water. The aeration process was performed using a constant flow diffusion system. The tests were carried out until the saturation of dissolved oxygen in each of the water samples was obtained.
Results:
The results show that the presence of clays during the transfer of DO have little influence on bodies of water. However, variations were observed in time intervals of <5 min in which the increase in the percentage of clays favored the transfer of DO.
Conclusions:
With these results, it is concluded that the presence of clays can generate a beneficial effect during the transfer of DO in bodies of water with a high oxygen deficiency, whereas, for water samples with a relatively high percentage of DO, clays can hinder the DO restoration process.
Keywords:
Dissolved oxygen (DO), diffuse aeration systems, clays, anoxic water..Resumen
Contexto:
La carencia de oxígeno disuelto (OD) en cuerpos de agua se ha convertido en un problema ambiental para varios ecosistemas debido a cambios drásticos en sus condiciones climáticas, bilógicas y físicas. La presencia de microorganismos, materia orgánica y arcillas, transportados por deslizamientos y aguas lluvias, afectan los procesos de restauración y transferencia de OD en el agua, lo que hace necesario estudiar cómo dichos factores afectan el proceso de transferencia de OD.
Método:
Este trabajo se centra en estudiar la influencia de las arcillas durante el proceso de transferencia de OD en cuerpos de agua. Para esto, se utilizaron muestras de arcillas de la región de Paipa-Boyacá, adicionadas en 1, 3 y 5% en peso a muestras de agua limpia. El proceso de aireación se llevó a cabo utilizando un sistema de difusión de flujo constante. Los ensayos se realizaron hasta obtener la saturación de oxígeno disuelto en cada una de las muestras de agua trabajadas.
Resultados:
Los resultados muestran que la presencia de arcillas durante la transferencia de OD tienen poca influencia en los cuerpos de agua. Sin embargo, se observaron variaciones en intervalos de tiempo <5 min en los que el incremento del porcentaje de arcillas favorecía la transferencia de OD.
Conclusiones:
Con estos resultados se concluye que la presencia de arcillas puede generar un efecto benéfico durante la transferencia de OD en cuerpos de agua con una alta carencia de oxígeno, mientras que, para muestras de agua con un porcentaje relativamente alto de OD, las arcillas perjudican el proceso de restauración de OD.
Palabras clave:
Oxígeno disuelto (OD), sistemas de aireación difusa, arcillas, agua anóxica..Introduccion
The lack of dissolved oxygen (DO) in bodies of water (WBs) has badly affected various ecosystems [1]. This lack depends on the following aspects: the quality of water and physical, biological, and climatic factors, which may hinder the content of DO in water, thus affecting the life present in WBs [2]- [4]. To overcome this problem, several methods have been introduced for the aeration and restoration of DO in WBs, enabling the mineralization and elimination of most contaminants from water [5]- [7]. A prominent example of this is the management of aeration processes by mechanical and diffusion methods, which have allowed the transfer and regulation of DO in anoxic water.
Mechanical aeration systems have a low energy consumption compared to conventional diffusion systems [2]. However, the application of diffusion systems in aeration processes is widely accepted due to their efficiency in large BW [8]. On the other hand, the aeration processes and the treatment of BW are related to other factors such as microorganisms, organic matter, and sludge due to landslides, which transport the clays through the rainwater and that can affect the transfer processes of DO in WBs [9]. The DO in water bodies can vary due to different reasons, which may be natural or anthropogenic. The latter is related to polluting processes and the alteration of ecosystems with raw wastewater discharges. Wastewater, when discharged into water bodies, can decrease the concentration of dissolved oxygen (DO) [10], [11]. Under natural conditions, the effect of rain can cause landslides, which transport sediments and clays that can affect DO transfer processes [9]. This, in turn, is influenced by other factors such as: temperature [12], high levels of organic matter [13], depth, river turbidity, and runoff caused by rainfall [14]- [16]. These agents affect the transfer of oxygen in the water, thus increasing energy consumption during water treatment processes. This is why the elimination of some of these agents prior to performing an aeration process is preferred [8].
In several cases, the presence of clays in WBs favors the retention and elimination of elements. This is the case of adsorption heavy metals, present in WBs, due to their positive charge, thus allowing a lower energy consumption in downstream purification processes [17]. Moreover, the presence of clays can also contribute to the removal of microorganisms while increasing the oxygen content in WBs [18]. However, the aforementioned studies have not shown the influence that clays can have during DO transfer processes in clean BWs with an oxygen deficit. The objective of this work is to study the influence of clays present in the Paipa-Boyacá region in Colombia on the transfer of DO in samples of oxygen-deficient waters (anoxic waters) produced on a laboratory scale.
Materials and experimental details
Measurements were made on 12 L samples of clean water with 5 repetitions per sample, for a total of 20 trials. In prior tests, the samples were treated by distillation to ensure the elimination of biological and particulate material. The initial dissolved oxygen was measured by using a Hachflexi HQ30d US Pat (6912050) oximeter at a height of 2800 m.a.s.l and a pressure of 740 hPa. The oximeters were placed inside the assembly, as shown in Fig. 1. The DO data obtained in water samples as an initial parameter was 7,32 g·L-1. This parameter allowed establishing the total dissolved oxygen in the water samples using Eq. (1). All tests were carried out at a constant temperature of 17 °C, giving greater importance of the study to the influence of the presence of clays on the transfer of DO in water.
The elimination of DO in the water was done by adding sodium sulfite (Na2SO3), as presented in Eq. (2), and a catalyst cobalt chloride was used [6]:
Figura 1: Experimental set-up for performance evaluation of the diffusion system used in this study
where Na2 SO3 is the molecular weight of sodium sulfite required in [g] to remove all the dissolved oxygen present in water samples, O is the molecular weight of oxygen [g], WNa2 SO3 is the molecular weight of sulfite in one mole of Na2 SO3, WO2 is the total dissolved oxygen content in each of the water samples in g, O2i is the DO content [g·L-1], and V is the water volume [L]. This study used an additional 0,5% sodium sulfite during the process to ensure the total elimination of the DO present in each of the water samples.
The use of the sulfite technique for the elimination of DO in this study allows a stable control system for each of the treated samples. The application of sulfite favors the elimination of the DO present in water by through te formation of sulfate ions, which is described by Eq. (3). To accelerate the kinetics of the reaction, CoCl 2 was used, thus ensuring that this reagent does not influence DO transfer [6]
The anoxic water samples were prepared with bentonite clays obtained from the study area. The study was carried out by adding 0, 1, 3 and 5% of the additional weight of bentonite in the anoxic water samples, making 3 replicates for each type of sample. The samples were stirred for 20 minutes to ensure the homogeneity of the clays in the solution, as well as the removal of clusters from the clay during deposition into the water samples. 5 replicates were made for each type of sample, for a total of 20 samples in this study.
The clay samples were obtained in the municipality of Paipa, Boyacá, Colombia. The material was sieved at 74 microns (200 meshes) and dried in an electric oven at 105 °C for 6 hours. To characterize the clay samples, they were homogenized and analyzed using the X-ray diffraction (XRD) technique with a Phillips PW1700 X-ray diffractometer, equipped with a 26° graphite monochromator, a Co source at wavelength λ= 1,75 Å, a PW1825 generator, and a Pixel variable angle detector with the Bragg-Brentano configuration [19]. The diffraction pattern is showed in Fig. 2, as well as the result of the clay composition analysis obtained through XRD. The characterization of the sample was performed using the HighScore-Plus software, supplied by PANanalytical X’pert Pro. The samples have a main composition of montmorillonite and illite (~79% of the total composition), which may be closely related and can contain other compounds in a lesser proportion, such as quartz and other silicate-type minerals [20], [21]. This study found that the clay samples used are composed of quartz, albite, and faujasite occurrents from the zone [22]. The XRD pattern shows a characteristic diffraction peak for quartz at an angle of 31°. The diffraction peaks generated for montmorillonite and illite are consistent with those reported by [23] using a source of CuK∝1 L3 (λ= 1,540598 Å). The transfer of the diffraction peaks is due to the type of source used.
The DO transfer process was carried out through a diffuse vertical bed aeration system, using a 16 W air pump with a 2,5 L·min-1 air transfer and a bubble membrane. The management of this type of aeration systems allows a better control of the variables that occur during theWBrestoration and DO transfer processes. Restricting operations to a system with vertical aeration, such as the one presented in Fig. 1, gives greater control over the DO transfer process, which allows to eliminate variations in the data due to the horizontal currents or flows within each system [24]. The tests were performed until a constant DO content was obtained. The data obtained in the DO transfer process for each of the measurements allowed determining the energy consumption and the efficiency of the aeration process using the calculations reported by [8]
Figura 2: XRD patterns of clays from the area of Paipa, Boyacá, Colombia, and used in this study
Results
The results obtained in this research are presented in the form of graphs for an optimal relation of the data obtained from each one of the analyzed samples. These graphs allowed us to observe the characteristics and behavior of the samples during the DO transfer process in each of the tests performed. The data obtained in this study were averaged, presenting a hatch of standard variation of ~0,5% on the contribution of DO in the water samples for all the studied cases.
The behavior of the DO transferred in each of the water samples worked as a function of time, as shown in Fig. 3. This process can be presented in the form of the percentage of DO shrinkage ( %) until it reaches the saturation point (Fig. 3a), or in the form the percentage of DO transfer ( %) supplied in each of the time intervals (Fig. 3b).
Figura 3: Behavior of the DO transferred in each of the water samples as a function of time (a) DO contraction percentage ( %) and b) percentage of DO transferred per time unit)
Analysis
The behavior of the DO in the 4 studied types of water samples during the aeration process is shown in Fig. 3. Fig. 3a shows the DO concentration as a function of time up to a stable DO value. The contribution of DO transfer within time intervals is shown in Fig. 3b. Other factors that have an influence during DO transfer in water, such as temperature, were not considered.
A stagnation zone of DO content occurs during the first 5 minutes of the aeration process. This behavior is reported by all the analyzed samples. The generated transfer stagnation is due to the application of an excess of sodium sulfite, which has been mentioned previously, thus guaranteeing the total removal of the oxygen present in the initial sample [25]. No variation is observed in the samples within this range, which rules out the influence of clays.
Similarly, a behavior in the DO transfer is presented in Fig. 3a for the 4 samples. Slight variations in samples were observed between the 5-10 and the 18-24-minute intervals. These areas are best visualized in Fig. 3b, where abnormal behavior can be observed. A greater contribution to the DO transfer rate was observed due to the increase in the content of clays in the water samples, thus obtaining a maximum peak of 17,5% of the total DO transferred for the sample with an addition of 5% of the clays present in the region of Paipa, Boyacá. This transfer peak decreased as the content of clays in the water was reduced, obtaining values of 17, 15,2, and 12,1% of DO, respectively, for the samples with 3, 1, and 0% (free of clays). The possible affinity between oxygen and particles can improve the transfer rate of DO in water [26].
The behavior of the process of transfer and saturation of DO in water samples in this study could be compared with the studies realized by [5]. This behavior is of the Arrhenius type, in which the oxygen content in the sample will gradually approach without reaching the maximum theoretical value. This behavior is also seen in the DO content with respect to the variation of the temperature, evincing an inverse behavior to that of the DO transfer. [27] reported the reduction of the DO transfer rate from the 10th minute of measurement and a saturation point from the 28th, obtaining a maximum saturation value of 95 ± 1,2% for each of the 4 studied samples. The decrease in the contribution rate of DO in the water samples from values close to the saturation point has also been reported by [28] and [29], who reported that the presence of high DO values, i.e., with a distance less than the maximum point of saturation or stagnation, which requires a greater amount of input time and of oxygenation in the water to reach this saturation point.
The data presented in Fig. 3b shows that the addition of and increase in the percentage of clays in the water samples does not exert a significant influence on the maximum saturation point and the DO transfer time. However, a slight decrease in DO concentration was observed between 16-20 minutes of measurement due to the addition and increase of clays in the water samples. This behavior was observed after reaching a DO concentration. The measurements were made in controlled environments. Therefore, they are not fully reproducible on a real scale in natural water bodies. There is a hatch of variables, such as temperature, dissolved metals, microorganisms, and so on, which also affect the DO transfer process in the water. However, the obtained results are presented as an initial stage prior to the study of the effect of these clays in environments outside laboratory conditions, as well as the study of other factors that affect water quality and DO content in water. These variables are expected to be analyzed in future research.n of 80 ± 5% (Fig. 3a). Increasing the suspended clay particles can reduce the total transfer area, thus generating a decrease in the contribution of DO at this stage of the process, i.e., generating a surface contamination that allows the contribution of DO to the WB [30].
The value of the coefficient at room temperature of the oxygen transfer (K La·h-1) shows an average value of 12,45 ± 3,28 a.u.With this value, it is possible to obtain an average value of N of 0,86 ± 0,12 kg O2·kW-1·h-1 for each of the samples in this study. This value remains within the range of efficiency in the aeration processes by diffusion mechanisms (from 0,3 to 1,2 kg O2·kW-1) [8], showing that the system behaves in conditions similar to those found at the industrial level. The values of the standard oxygen transfer rate (SOTR) and standard oxygen transfer efficiency (SOTE) are 12,31 kg O2·h-1 with an efficiency of 38,48 %. Therefore, the energy consumption of each of the samples remained within the range established by the literature [8], [31], [32]. These values can vary with pressure generated by the flow of the gas that transports oxygen, as well as the height of the water column (depth) [33]. This opens a study hatch to analyze the effect of these parameters in future work.
From a general point of view, the study shows the following:
-
the presence of clays in anoxic water bodies favors the required DO transfer;
-
the presence of clays in water bodies with a lower DO content, but close to its saturation point in the water, shows a harmful behavior in reducing the DO transfer capacity in water; and
-
the variation and increase of clays in cases 1) and 2) affects the processes of DO transfer.
This is favorable for water bodies lacking DO (anoxic), while a low or null content of clays in bodies of water with a relatively high DO content but it produces a lower than the saturation point of the DO in water. Our results are consistent with previously reported work [34], which presented the low influence of clays during the transfer of DO in bodies of water. The presence of clays exerts a degree of influence on the DO transfer process in a superficial way.
These measurements were made on a laboratory scale, so they should be taken as an initial phase that must be tested with other variables that affect DO concentration, considering the characteristics of the natural water bodies to be studied. Factors such as temperature were not considered in this study; we focused only on the effect of the clay studied during the transfer of DO in anoxic water samples under controlled conditions.
Conclusions
In this study, we have shown the possible influence of the clays on WBs during DO transfer processes in a controlled environment. The data obtained in this study allowed us to establish that the presence of clays in WBs with a lack of DO (anoxic waters) does not have a direct influence on the DO saturation point during the oxygen transfer process through the use of diffuse aeration, i.e., the presence and increase of the concentration of clays present in Paipa, Boyacá, Colombia. However, the data allowed us to establish that the presence of clays in the water can favor the transfer of oxygen in the first periods of time. Therefore, this is an energy advantage for WBs with a high lack of DO and a relatively high percentage of clays. The behavior decreases as the DO content in the system increases, with a low clay content within this type of WB, which promises to be a better option.
The measurements were made in controlled environments. Therefore, they are not fully reproducible on a real scale in natural water bodies. There is a hatch of variables, such as temperature, dissolved metals, microorganisms, and so on, which also affect the DO transfer process in the water. However, the obtained results are presented as an initial stage prior to the study of the effect of these clays in environments outside laboratory conditions, as well as the study of other factors that affect water quality and DO content in water. These variables are expected to be analyzed in future research.
Referencias
License
Copyright (c) 2020 Nicolas Rojas-Arias, Cesar René Blanco Zuñiga, Ludy Yiseth Peña Pardo, Martín Emilio Mendoza Oliveros, Segundo Agustín Martínez Ovalle

This work is licensed under a Creative Commons Attribution-NonCommercial-ShareAlike 4.0 International License.
From the edition of the V23N3 of year 2018 forward, the Creative Commons License "Attribution-Non-Commercial - No Derivative Works " is changed to the following:
Attribution - Non-Commercial - Share the same: this license allows others to distribute, remix, retouch, and create from your work in a non-commercial way, as long as they give you credit and license their new creations under the same conditions.